Physicists Record Inaugural Sounds of Heat ‘Sloshing’ in a Superfluid, Unveiling Wave-like Heat Movement
Heat typically scatters in most materials, gradually fading as it warms its surroundings. However, in certain rare states of matter, heat can behave like a wave, similar to how sound waves bounce from one end of a room to the other. This wave-like heat is referred to as “second sound” by physicists.
Only a few materials have shown signs of second sound. Recently, physicists from MIT have successfully captured direct images of second sound for the first time.
These new images demonstrate how heat can move in a wave-like manner, sloshing back and forth, even when the physical matter of the material moves differently. The images showcase the pure movement of heat, independent of the material’s particles.
To provide an analogy, Assistant Professor Richard Fletcher explains, “Imagine having a tank of water where one half is almost boiling. If you observe the water, it may appear completely calm, but suddenly one side becomes hot, then the other side becomes hot, and the heat continues to move back and forth, while the water remains seemingly still.”
Under the guidance of Martin Zwierlein, the Thomas A Frank Professor of Physics, the team visualized second sound in a superfluid. A superfluid is a unique state of matter that forms when a cloud of atoms is cooled to extremely low temperatures, causing the atoms to flow like a fluid without any friction. In this superfluid state, theorists have predicted that heat should also flow as a wave, but until now, scientists had not been able to directly observe this phenomenon.
The recent findings, published in the journal Science, will provide physicists with a more comprehensive understanding of how heat is transferred in superfluids and other related materials, such as superconductors and neutron stars.
According to Zwierlein, there are significant correlations between our extremely thin gas, which is a million times thinner than air, and the behavior of electrons in high-temperature superconductors, as well as neutrons in incredibly dense neutron stars. He further explains that this breakthrough allows us to examine the temperature response of our system in a pristine manner, offering insights into concepts that are otherwise challenging to comprehend or explore.
Zwierlein and Fletcher collaborated with several co-authors on this study, including Zhenjie Yan, a former physics graduate student who served as the first author, as well as former physics graduate students Parth Patel and Biswaroop Mikherjee. Additionally, Chris Vale from Swinburne University of Technology in Melbourne, Australia, contributed to the research. It is worth noting that the MIT researchers are affiliated with the MIT-Harvard Center for Ultracold Atoms (CUA).
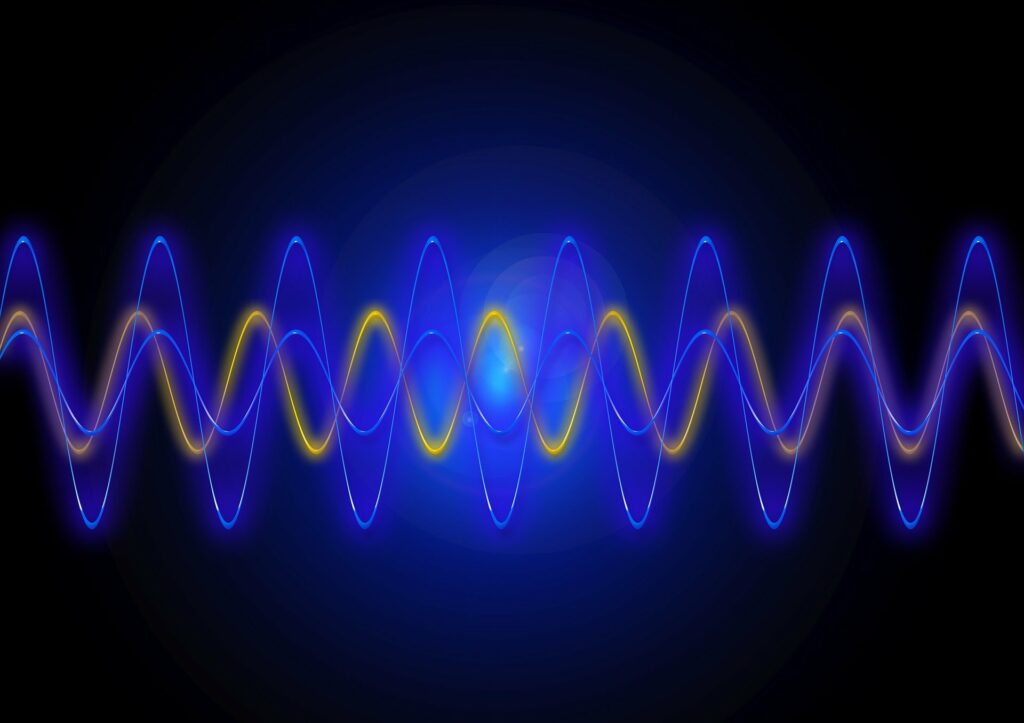
Super sound
When temperatures are lowered to near absolute zero, clouds of atoms can undergo a transformation into rare forms of matter. At MIT, Zwierlein’s research group is investigating the unique phenomena that arise among ultracold atoms, particularly fermions – particles like electrons that typically repel each other.
Under specific conditions, fermions can be induced to strongly interact and form pairs. In this paired state, fermions exhibit unconventional flow behavior. For their most recent experiments, the team utilizes fermionic lithium-6 atoms, which are trapped and cooled to temperatures in the nanokelvin range.
In 1938, physicist László Tisza proposed a two-fluid model to explain superfluidity, suggesting that a superfluid is a combination of a normal, viscous fluid and a frictionless superfluid. According to this model, the mixture of these two fluids should support two types of sound: ordinary density waves and unique temperature waves, later termed “second sound” by physicist Lev Landau.
Considering that a fluid transitions into a superfluid at a specific ultracold temperature, the MIT team hypothesized that the two types of fluid should also conduct heat differently. In regular fluids, heat dissipates as expected, while in a superfluid, it can propagate as a wave, similar to sound.
“Second sound is a defining characteristic of superfluidity, but in ultracold gases, it has only been observed indirectly through the accompanying density ripples,” explains Zwierlein. “The nature of the heat wave could not be definitively established until now.”
Tuning in
Zwierlein and his team aimed to isolate and observe second sound, the wave-like movement of heat, without the interference of fermions’ physical motion in their superfluid. To achieve this, they developed a novel thermography technique called heat-mapping. In typical materials, infrared sensors are used to visualize heat sources.
However, at extremely low temperatures, gases do not emit infrared radiation. Instead, the team devised a method to utilize radio frequency to detect the movement of heat in the superfluid. They discovered that lithium-6 fermions resonate at varying radio frequencies depending on their temperature. When the cloud contains more normal liquid at higher temperatures, it resonates at a higher frequency. Conversely, colder regions in the cloud resonate at a lower frequency.
By applying the higher resonant radio frequency, any normal fermions in the liquid responded by emitting a ringing sound. The researchers were then able to precisely identify and track these resonating fermions over time, generating “movies” that unveiled the pure motion of heat, resembling the back and forth sloshing of sound waves.
Zwierlein states, “For the first time, we can visually capture this substance as we cool it below the critical temperature of superfluidity and directly witness its transition from a mundane normal fluid, where heat equilibrates uniformly, to a superfluid where heat oscillates back and forth.”
These experiments represent the initial instance in which scientists have directly imaged second sound and the pure motion of heat in a superfluid quantum gas.
The researchers intend to expand their investigations to more accurately map the behavior of heat in other ultracold gases. Subsequently, they believe their findings can be extrapolated to predict how heat propagates in other strongly interacting materials, such as high-temperature superconductors and neutron stars.
Zwierlein concludes, “Now, we will be able to precisely measure the thermal conductivity in these systems and aspire to comprehend and design more advanced systems.”
This article is republished from PhysORG under a Creative Commons license. Read the original article.
Do not forget to share your opinion with us to provide you with the best posts !
0 Comments