Scientists provide initial glimpse of electrons in motion in real-time within liquid water
In a study resembling stop-motion photography, scientists have successfully captured the movement of an electron while simultaneously immobilizing the much larger atom it orbits within a sample of liquid water.
These findings, which have been documented in the journal Science, offer a fresh perspective into the electronic structure of molecules in their liquid state, providing insights that were previously unattainable using X-rays. This innovative technique allows for the immediate observation of the electronic response when an object is exposed to X-ray radiation, thereby aiding in the comprehension of the impact of radiation on both objects and individuals.
Linda Young, a senior author of the research and Distinguished Fellow at Argonne National Laboratory, explained, “The chemical reactions triggered by radiation that we aim to investigate are a direct consequence of the target’s electronic response, which occurs within an attosecond timeframe.” She further added, “Previously, radiation chemists could only analyze events occurring on a picosecond timescale, which is a million times slower than an attosecond. It’s akin to saying ‘I was born and then I died.’ The details of what transpires in between are what we can now uncover.”
To achieve these breakthroughs, a collaborative team of scientists from various Department of Energy national laboratories and universities in the United States and Germany combined experimental data with theoretical models. This allowed them to observe, in real-time, the repercussions when ionizing radiation from an X-ray source interacts with matter.
The research team will gain a deeper understanding of complex radiation-induced chemistry by focusing on the time scales in which the action occurs. Initially, these researchers collaborated to create the necessary tools for comprehending the impact of prolonged exposure to ionizing radiation on the chemicals present in nuclear waste.
According to Carolyn Pearce, a PNNL chemist and the director of IDREAM EFRC, members of our early-career network actively participated in the experiment and subsequently joined our complete experimental and theoretical teams to analyze and comprehend the collected data. The invaluable IDREAM partnerships played a crucial role in our success.
From the Nobel Prize to the field
The actions of subatomic particles are so rapid that they can only be observed using a probe capable of measuring time in attoseconds. To put this into perspective, there are more attoseconds in a second than there have been seconds in the entire history of the universe. This groundbreaking research builds upon the field of attosecond physics, which was recognized with the 2023 Nobel Prize in Physics.
Attosecond X-ray pulses are only available in a few specialized facilities worldwide, and this particular study was conducted at the Linac Coherent Light Source (LCLS) in Menlo Park, Calif. The team at LCLS has been at the forefront of developing attosecond X-ray free-electron lasers. The researchers utilized a technique called X-ray attosecond transient absorption spectroscopy in liquids to observe the movement of electrons energized by X-rays before the atomic nucleus has a chance to react. Their chosen test case was liquid water. This innovative tool allows scientists to track the movement of electrons and witness the formation of newly ionized molecules in real-time. The implications of this research are vast and open up new avenues for attosecond science.
The recently disclosed discoveries effectively settle a longstanding scientific dispute regarding the origin of X-ray signals observed in prior experiments, whether they arise from distinct structural configurations or “motifs” of water or hydrogen atom dynamics. These experiments undeniably establish that such signals do not provide evidence for two structural motifs in the surrounding liquid water.
According to Young, “Essentially, the observations made in previous experiments were a result of the blurriness caused by the motion of hydrogen atoms. By conducting all our recordings prior to the atoms’ movement, we successfully eliminated this factor.”
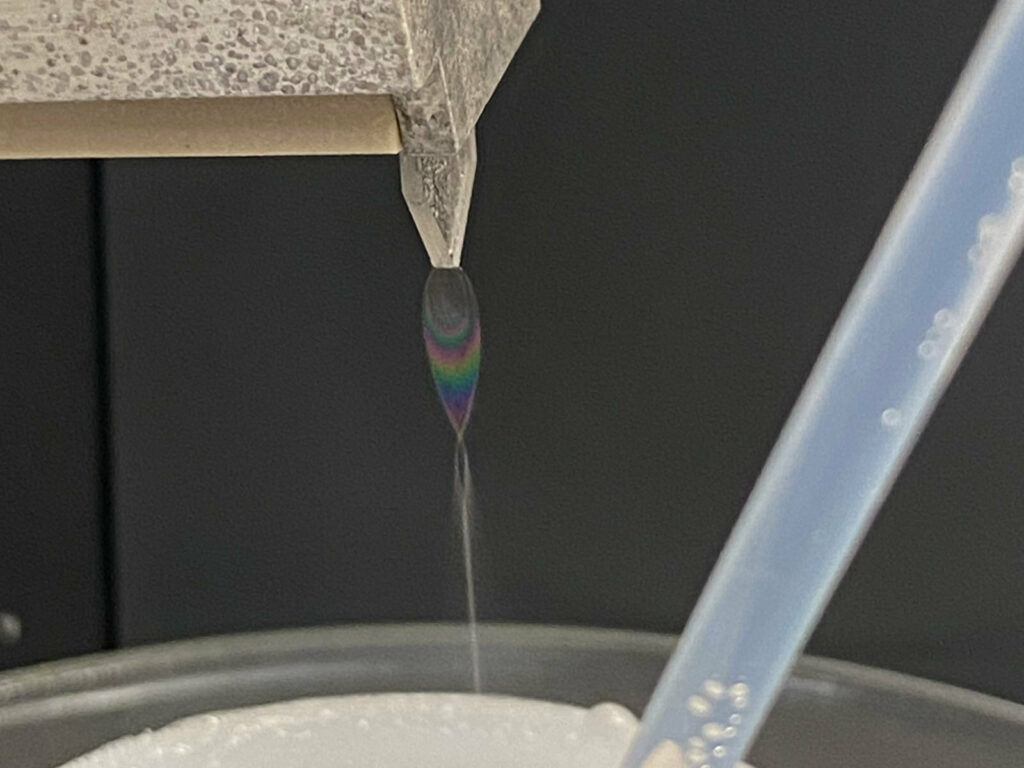
From simple to complex reactions
The current study is seen by the researchers as the commencement of a completely new direction for attosecond science. In order to make this discovery, experimental chemists from PNNL collaborated with physicists from Argonne and the University of Chicago, X-ray spectroscopy specialists and accelerator physicists from SLAC, theoretical chemists from the University of Washington, and attosecond science theoreticians from the Hamburg Center for Ultrafast Imaging and the Center for Free-Electron Laser Science (CFEL), Deutsches Elektronen-Synchrotron (DESY), in Hamburg, Germany.
Amidst the global pandemic in 2021 and extending into 2022, the PNNL team utilized techniques developed at SLAC to disperse an extremely thin layer of pure water along the path of the X-ray pump pulse.
“We required a smooth, thin sheet of water where we could concentrate the X-rays,” stated Emily Nienhuis, a young chemist at PNNL, who initiated the project as a post-doctoral research associate. “This capability was developed at the LCLS.” At PNNL, Nienhuis demonstrated that this method can also be employed to examine the specific concentrated solutions that are pivotal to the IDREAM EFRC and will be explored in the subsequent phase of the research.
From experiment to theory
After collecting the X-ray data, theoretical chemist Xiaosong Li and graduate student Lixin Lu from the University of Washington utilized their expertise in interpreting X-ray signals to replicate the signals observed at SLAC. The CFEL team, led by theoretician Robin Santra, simulated the response of liquid water to attosecond X-rays to confirm that the observed signal was indeed limited to the attosecond timescale.
Li, the Larry R. Dalton Endowed Chair in Chemistry at the University of Washington and a Laboratory Fellow at PNNL, stated, “We employed a state-of-the-art computational chemistry technique using the Hyak supercomputer at the University of Washington, allowing us to extensively characterize the transient high-energy quantum states in water. This breakthrough methodology significantly advanced our understanding of ultrafast chemical transformations at the quantum level, providing exceptional accuracy and atomic-level detail.”
Principal Investigator Young initiated the study and oversaw its execution, with first author and postdoc Shuai Li leading the on-site operations. Physicist Gilles Doumy from Argonne and graduate student Kai Li from the University of Chicago were also part of the team responsible for conducting the experiments and analyzing the data. Argonne’s Center for Nanoscale Materials, a DOE Office of Science user facility, contributed to characterizing the water sheet jet target.
Together, the research team gained insight into the real-time movement of electrons in liquid water while the rest of the world remained stationary.
“The methodology we developed allows for the investigation of the origin and progression of reactive species generated by radiation-induced processes, such as those encountered in space travel, cancer treatments, nuclear reactors, and legacy waste,” explained Young.
This article is republished from PhysORG under a Creative Commons license. Read the original article.
Do not forget to share your opinion with us to provide you with the best posts !
0 Comments