We’re on The Brink of Hearing The Universe’s Background Hum. Here’s Why We’re Listening
The cosmos ought to be in motion.
Gravitational waves might or should come from any supernova, neutron star or black hole merger, even a lone neutron star that is spinning quickly.
Even the Big Bang’s fast expansion of space 13.8 billion years ago should have generated a cascade of gravitational waves on its own.
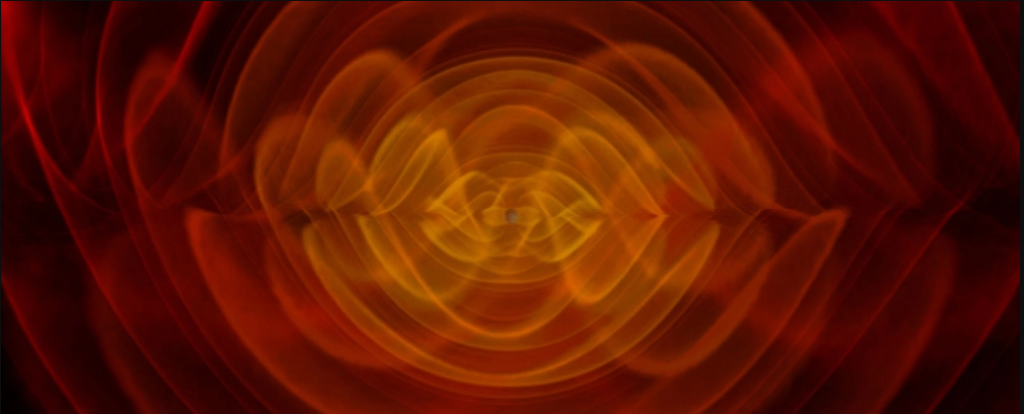
These enormous occurrences should cause ripples to reverberate through the very fabric of space-time, like a pebble tossed in a pond. These little expansions and contractions of space may be discernible to us as differences in what should be perfectly synchronized signals.
The gravitational wave background is one of maybe the most sought-after detections in gravitational wave astronomy. It is created when this variety of signals come together to generate a random or “stochastic” buzz.
The new frontier in space exploration
The discovery of the gravitational wave background is anticipated to completely change our knowledge of the universe and its development, much like the discovery of the cosmic microwave background did before it (and continues to do).
Theoretical physicist Susan Scott of the Australian National University and the ARC Centre of Excellence for Gravitational Wave Discovery says that finding a stochastic gravitational radiation background can reveal a wealth of data about astrophysical source populations and processes in the very early Universe that is not otherwise available.
“For instance, electromagnetic radiation cannot depict the Universe before the moment of the final scattering (about 400,000 years after the Big Bang). However, gravitational waves can provide us with information as far back as the beginning of inflation, which occurred about 10–32 seconds after the Big Bang.”
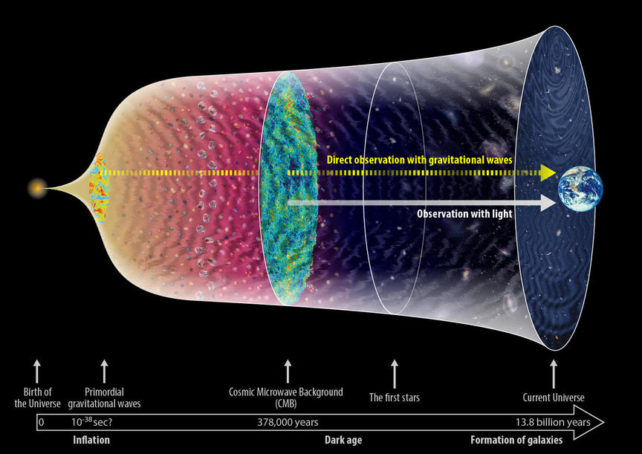
We should briefly discuss the cosmic microwave background, or CMB, another artifact of the Big Bang in order to comprehend the gravitational wave background’s significance.
The seething froth that was everything congealed into an opaque soup of subatomic particles known as ionized plasma moments after our Universe began to tick and space started to cool.
Any radiation that escaped alongside it was dispersed, preventing it from traveling very far. Light couldn’t travel freely through the universe or down through the eons until these subatomic particles recombined into atoms, a period known as the Epoch of Recombination.
Around 380,000 years after the Big Bang, the first flash of light exploded over space; throughout the next billions of years, as the Universe expanded, this light was drawn into every crevice. Today, it’s still all around us. Even though it is very weak, this radiation may be seen, especially at microwave wavelengths. The earliest light in the universe is the CMB.
Small temperature swings indicated by that initial light were the cause of the anomalies in this light, known as anisotropies. The CMB is one of our last remaining probes into the conditions of the early Universe, and its discovery cannot be overstated.
A wonderful reproduction of this accomplishment would be the finding of the gravitational wave backdrop.
As with the measurement of the cosmic microwave background and associated anisotropies, Scott claims that “we anticipate the detection and study of the gravitational wave background will alter our knowledge of the Universe.”
The buzz beyond the boom-crash
Just a few years ago, in 2015, gravitational waves were first detected.
Around 1.4 billion years ago, two black holes collided, sending forth waves that traveled at light speed. On Earth, these expansions and contractions of space-time very weakly activated an instrument that had been developed and perfected for decades in anticipation of such an occurrence.
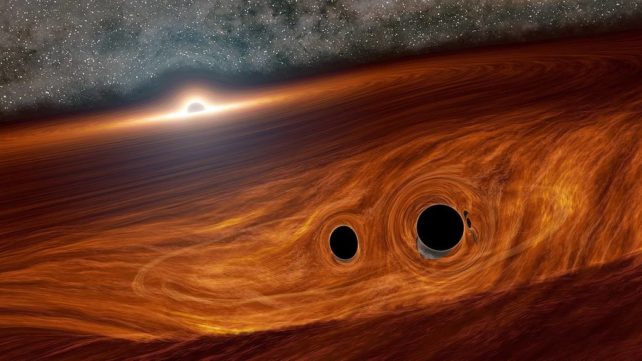
It was a huge discovery for a number of reasons. For the first time, it provided us with direct evidence of black holes’ existence.
It established the existence of gravitational waves, which was predicted by the General Theory of Relativity 100 years earlier.
And it meant that this long-awaited instrument, the gravitational wave interferometer, would fundamentally alter how we think about black holes.
And so it is. Nearly 100 gravitational wave events—those powerful enough to leave a noticeable signature in the data—have been discovered so far by the LIGO and Virgo interferometers.
These interferometers employ lasers that shine down unique, multi-kilometer-long tubes. The gravity waves’ effects on these lasers provide an interference pattern that allows researchers to deduce information about the characteristics of the compact objects that caused the signals.
The gravitational wave background, though, is a very other animal.
According to Scott, an astrophysical background is created by the jumbled noise of several weak, unrelated, and unresolved astronomical sources.
“Although the astrophysical background from stellar mass binary black hole mergers is anticipated to be a major source of the gravitational wave background (GWB) for this current generation of detectors, our ground-based gravitational wave detectors LIGO and Virgo have already detected gravitational waves from tens of individual mergers of a pair of black holes. We are aware that there are many of these mergers that are too many to resolve separately, and when they combine, they cause the detectors to vibrate with random noise.”
Although the rate of binary black hole collisions in the universe is unknown, we may approximate it from the pace at which we can find them.
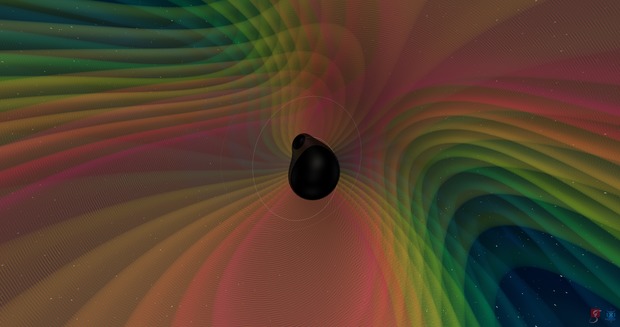
Scientists estimate that there are between one and several mergers every hour, with the visible signal from each lasting only a brief period of time. Astrophysicists liken the background noise produced by these individual, random signals, which would be too faint to be detected, to the sound of popping popcorn.
With tools like the LIGO and Virgo interferometers, we may anticipate discovering a stochastic gravitational wave signal from this source. These equipment are now receiving upkeep and preparation, and in March 2023, a new observation cycle will include them in addition to a third observatory, KAGRA in Japan. It is possible that this teamwork will find the popcorn GWB.
However, there are other items in the gravitational wave toolbox as well. And other instruments will be able to find other gravitational wave background sources. The Laser Interferometer Space Antenna (LISA), whose launch is scheduled for 2037, is one such instrument that is still 15 years away.
With “arms” that are 2.5 million kilometers long, it uses the same technology as LIGO and Virgo. It will function at a considerably lower frequency than LIGO and Virgo, allowing it to pick up various gravitational wave occurrences.
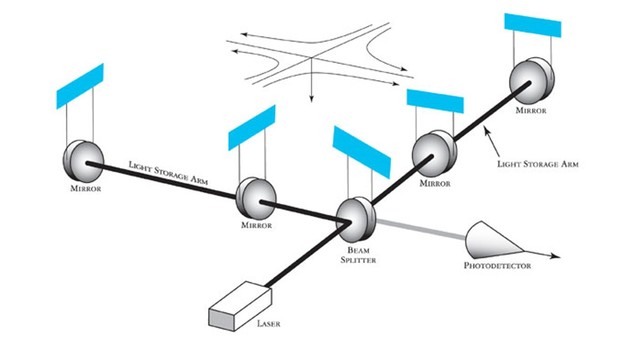
According to Scott, “The GWB is not always popcorn-like.”
“It may also be made up of discrete deterministic signals that cross over at different points in time to create confused noise, much like the background chatter at a party. The gravitational radiation generated by the population of compact white dwarf binaries in our galaxy is an illustration of confused noise. For LISA, this will be a significant source of confusing noise. When trying to identify other weak gravitational wave transmissions in the same frequency range, the stochastic signal in this scenario is so powerful that it forms a foreground, adding to the noise.”
Theoretically, LISA might also find cosmological sources of gravitational waves, such as cosmic inflation immediately following the Big Bang or cosmic strings, which are hypothetical universe fissures that may have formed at the conclusion of inflation and may have lost energy through gravitational waves.
Timing the pulse of the cosmos
Pulsar timing arrays are another massive gravitational wave observatory that researchers have been examining to search for signs of the gravitational wave background. The remnants of once-massive stars that died in a spectacular supernova, leaving just a dense core left, are known as neutron stars, including the kind of object known as pulsars.
Some pulsars revolve in such a way that radio emission beams from their poles sweep across Earth like a cosmic lighthouse; this property is valuable for a variety of purposes, including navigation.
However, theoretically, minute abnormalities in the timing of pulsar flashes should result from the stretching and tightening of space-time.
Small time discrepancies in a single pulsar would not indicate much, but if several pulsars had connected timing discrepancies, that might be a sign of gravity waves coming from inspiraling supermassive black holes.
Pulsar timing arrays may provide tempting signs of this source of the gravitational wave background, but there is not yet enough information to confirm it.
We are tantalizingly close to detecting the gravitational wave background, which includes the cosmological background, which includes the quantum fluctuations seen in the CMB, inflation, and the Big Bang itself, as well as the astrophysical background, which reveals the behavior of black holes throughout the Universe.
The one we won’t see until the challenging task of disentangling the backdrop into the separate components that make up the noisy whole, according to Scott, is this.
The discovery of gravitational waves from the Big Bang, she adds, is actually the ultimate objective of gravitational wave astronomy. “While we look forward to a lot of information coming from the detection of an astrophysically created backdrop,” she says.
The proposed third generation ground-based detectors, like the Einstein Telescope and Cosmic Explorer, “could be sensitive to a cosmologically produced background with 5 years of observations, thereby entering the realm where important cosmological observations can be made” by eliminating this binary black hole foreground.
Do not forget to share your opinion with us to provide you with the best posts !
0 Comments